Sequence Optimization
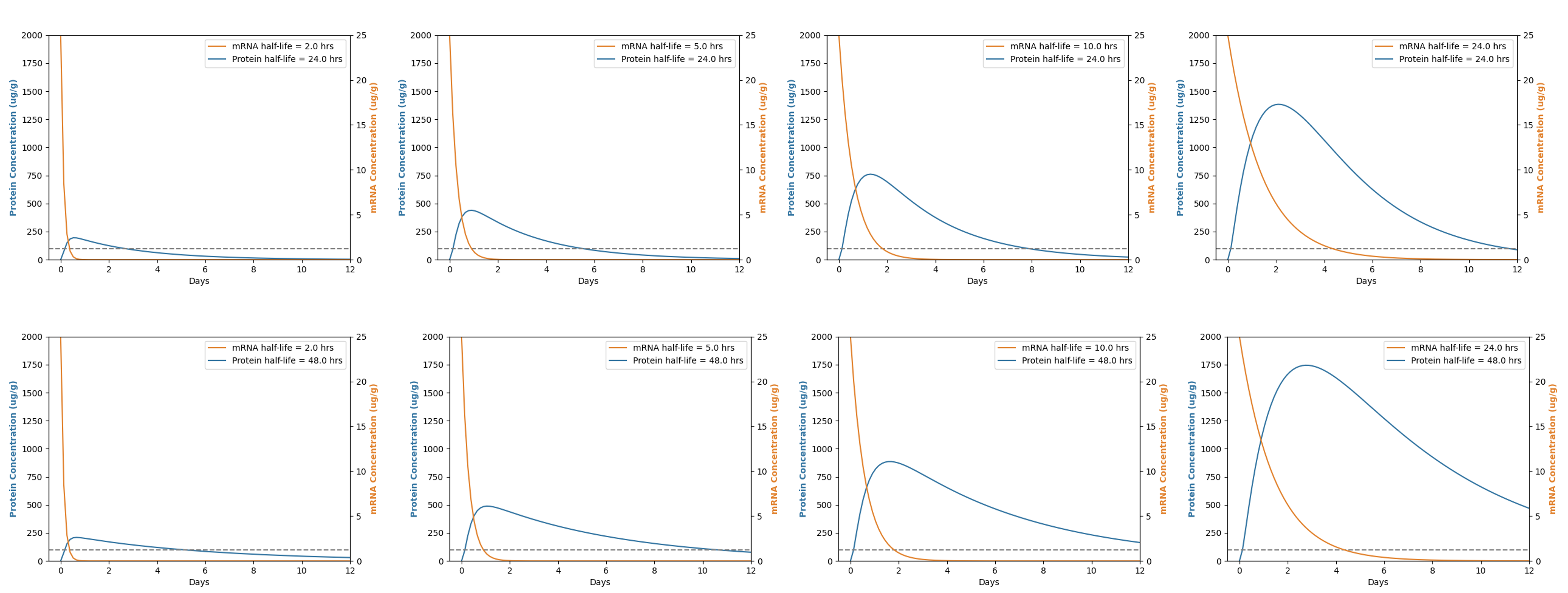
The half-life of mRNA and the half-life of the protein jointly determine the expression levels and duration of the protein within the cell. A longer half-life of mRNA enables its encoded proteins to be utilized for a more extended period by the cellular translational machinery, thereby increasing the overall protein yield. A longer half-life of the protein signifies a slower rate of degradation within the cell, thus extending the time it remains in a functional state.
If both the mRNA and the protein possess long half-lives, this leads to sustained high levels of protein expression within the cell. The stability of mRNA ensures ample time for protein synthesis, whereas the long half-life of the protein guarantees that once synthesized, they will retain their activity for an extended period within the cell. Consequently, for proteins that require continuous and stable expression, design considerations should include enhancing both the half-life of the mRNA and the half-life of the protein. This dual strategy facilitates the maintenance of steady-state protein levels necessary for sustained cellular function.
mRNA Sequence Optimization
mRNA serves as the critical intermediary for the transmission of genetic information from DNA to the protein synthesis mechanism. Its expression and stability are influenced by a variety of factors, including but not limited to the structural features of the mRNA, its sequence composition, the intracellular environment, and the protein factors that interact with it.
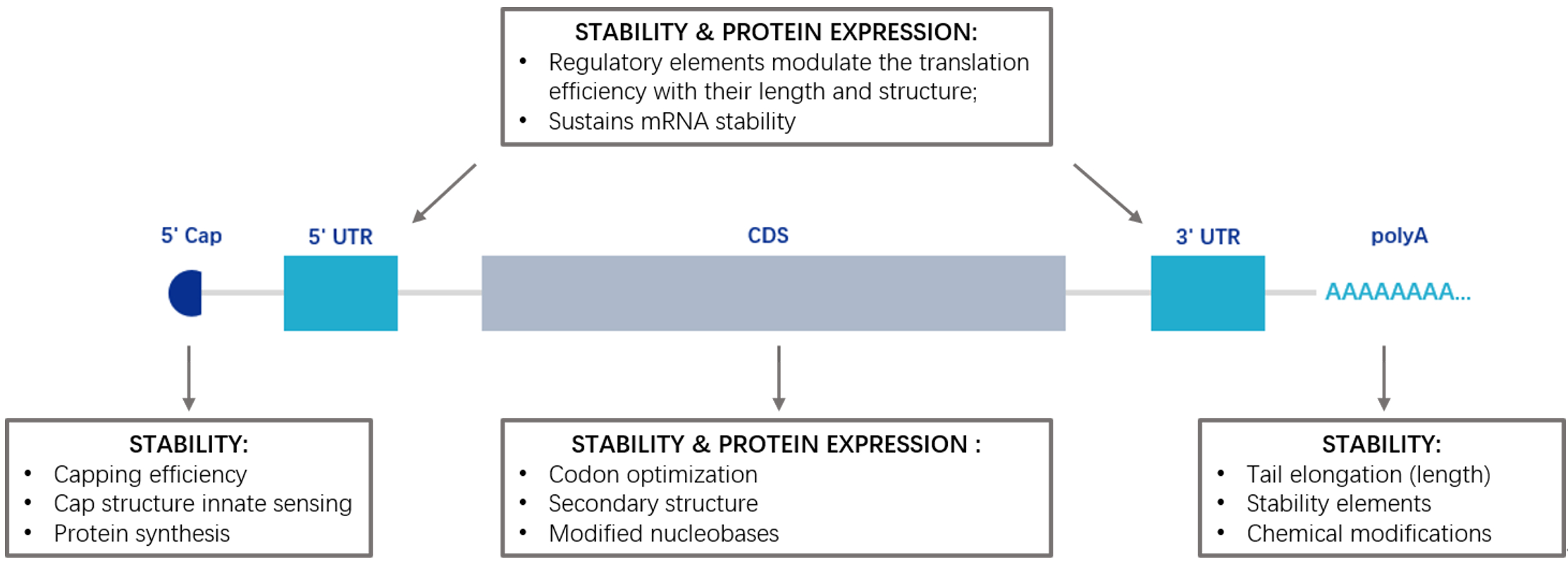
Optimization of mRNA
In our research, we conducted a comprehensive analysis and optimization of various factors affecting mRNA expression efficiency and stability through advanced bioinformatics methods and molecular biology techniques.
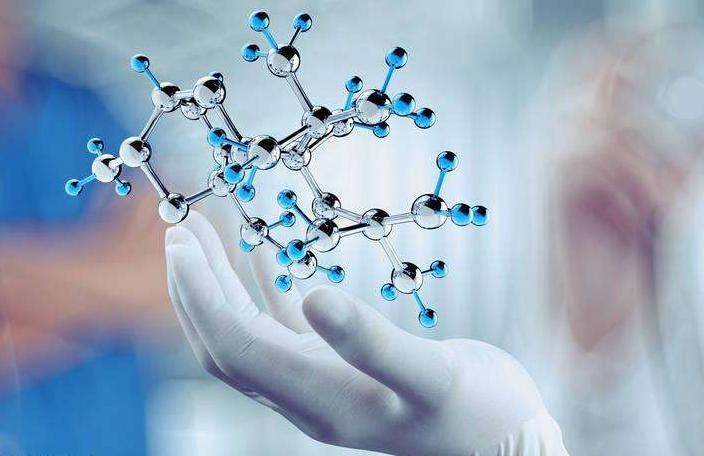
5' Cap
The 5' cap structure contains a 7-methylguanosine nucleoside, which can regulate mRNA splicing, protect mRNA from degradation by exonucleases, and regulate mRNA translation through the eIF4F complex formed with translation initiation factors.
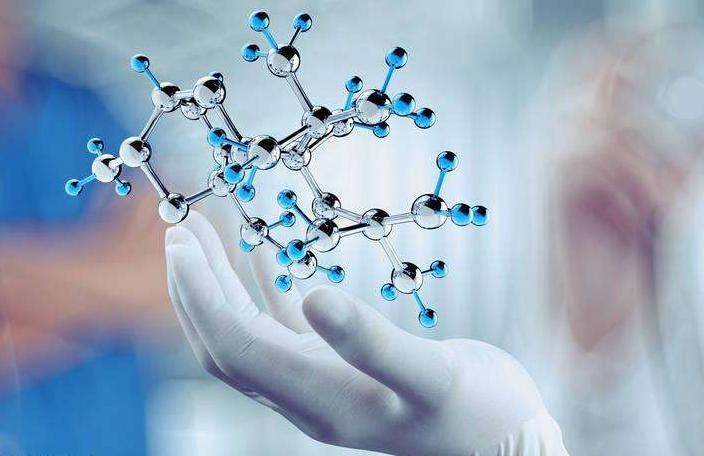
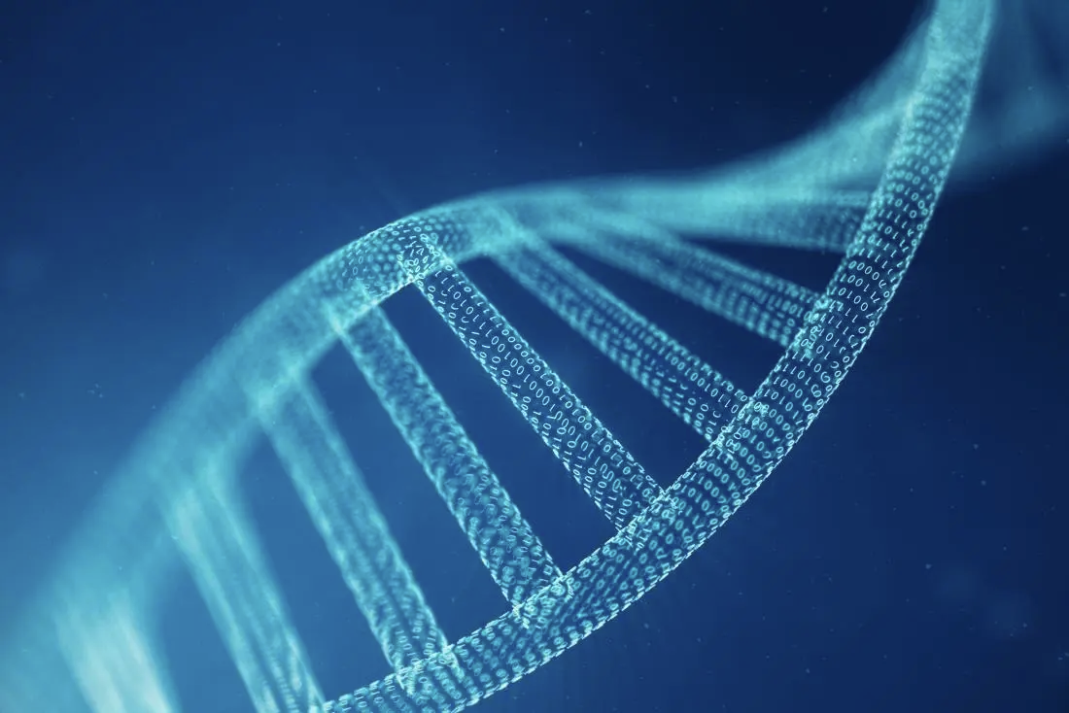
UTR' 5
The 5' UTR (5' untranslated region) is located at the 5' end of mRNA, preceding the start codon (typically AUG). This region plays a crucial role in translation initiation. Optimization strategies include preventing the formation of secondary structures and reducing stable structures near the start codon to enhance translation efficiency.
CDS
Translation of mRNA is a complex process involving the interaction of multiple molecules. In this process, the mRNA is first recognized and bound by the ribosome. The ribosome then moves along the mRNA, sequentially recognizing and translating the codons on the mRNA. Finally, translation stops when a stop codon is encountered, and the newly synthesized protein is released. For mRNA, optimizing the coding sequence (CDS) is a crucial task as it directly impacts translation efficiency and protein yield. The primary goal of CDS optimization is to enhance mRNA translation efficiency, thereby increasing protein production.
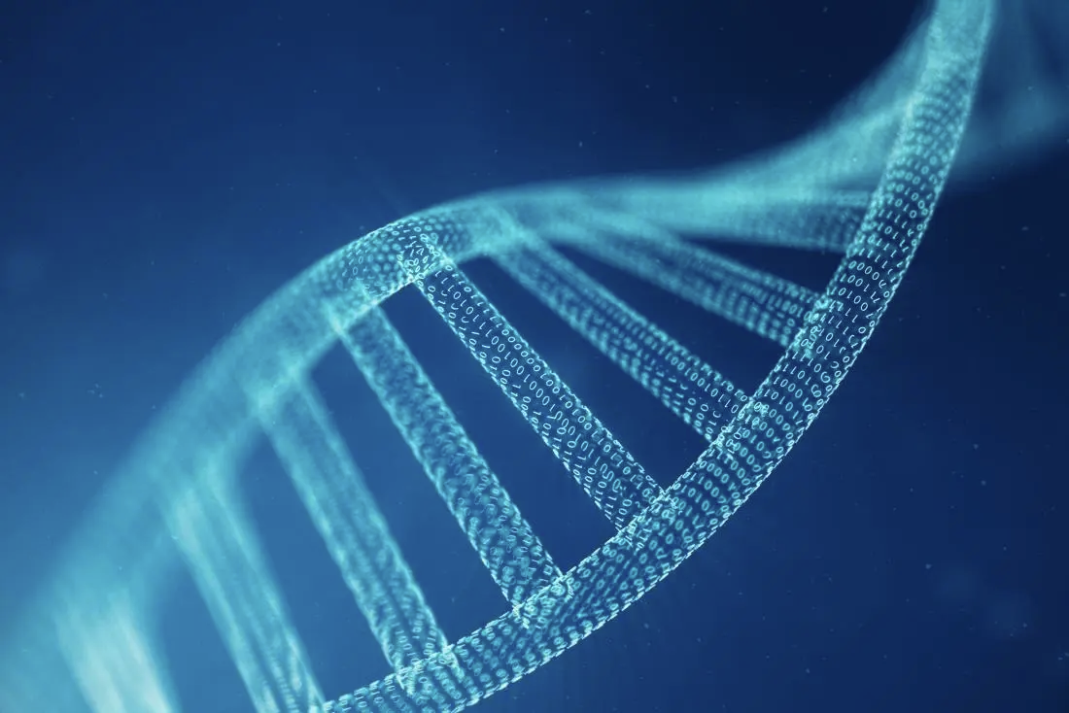
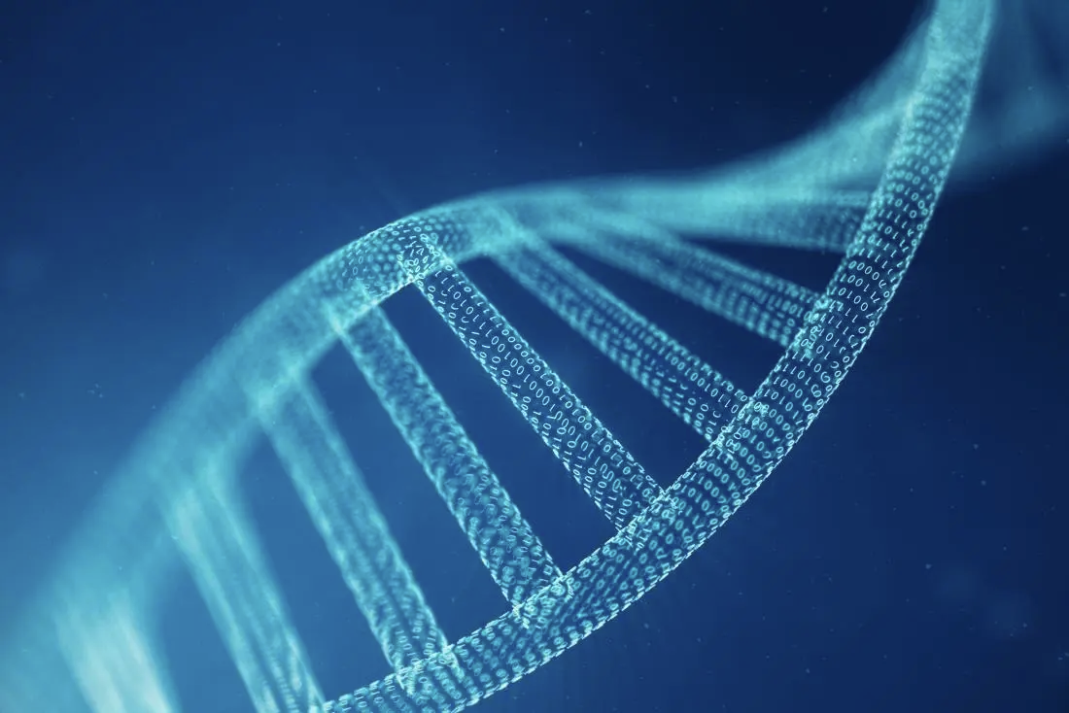
UTR' 3
The 3' UTR (3' untranslated region) is located at the 3' end of mRNA, following the stop codon. It plays a significant role in mRNA stability and translation efficiency. Optimization strategies involve removing unstable elements and adding stable elements to improve mRNA stability.
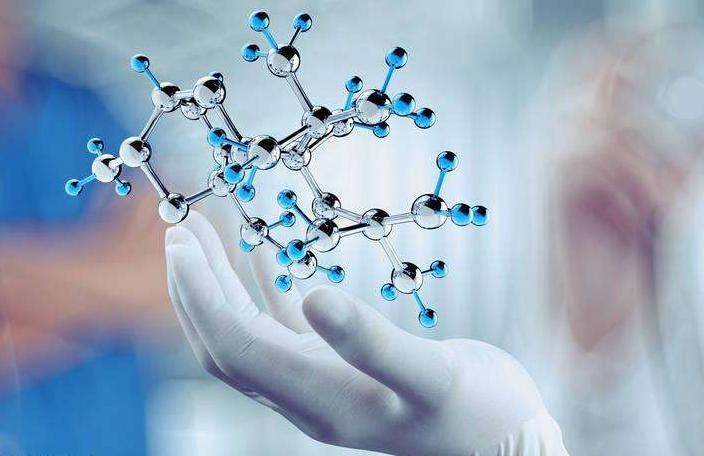
poly A
The poly A tail is a string of adenine nucleotides added to the 3' end of mRNA. It typically consists of 50-250 adenine nucleotides, but the length can vary depending on the mRNA and the organism. The poly A tail plays a crucial role in the lifecycle of mRNA, significantly influencing mRNA stability, nuclear-cytoplasmic transport, and translation efficiency.
Protein Sequence Optimization
Protein design can be used to enhance the stability of therapeutic proteins, increase the heat resistance of industrial enzymes, optimize the specificity of biosensors, and provide personalized solutions for biotherapies. In the process of protein design, enhancing expression levels and stability is one of the core objectives. Higher expression levels mean that more proteins can be obtained in the production process, thereby reducing costs and improving production efficiency. At the same time, enhancing protein stability allows it to maintain function under a wider range of environmental conditions, extend its lifespan both inside and outside the body, thereby improving its application range and efficacy.
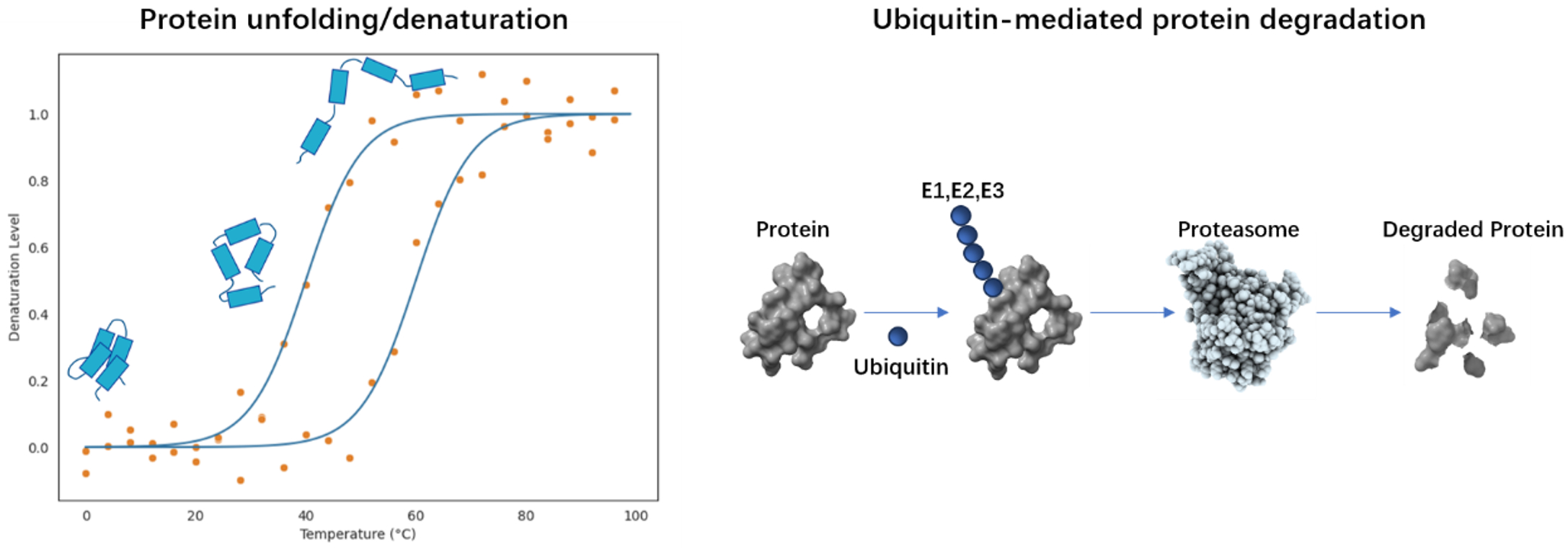
Stratergy 1: Enhancing Thermal Stability of Proteins
High temperatures or heat stress can cause protein denaturation, leading to loss of function. In living organisms, this situation typically triggers a stress response, such as the production of heat shock proteins, to assist in restoring the protein to its normal structure.
The main strategies to improve the thermal stability of proteins include:
- 1. Increasing hydrophobic amino acids.
- 2. Increasing ion bonds and hydrogen bonds.
- 3. Introduction of disulfide bonds.
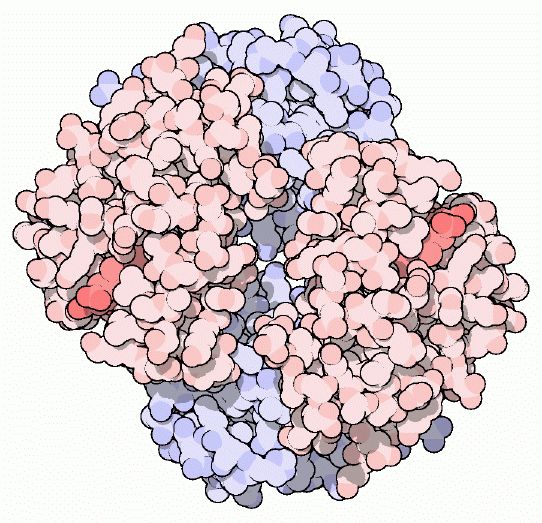
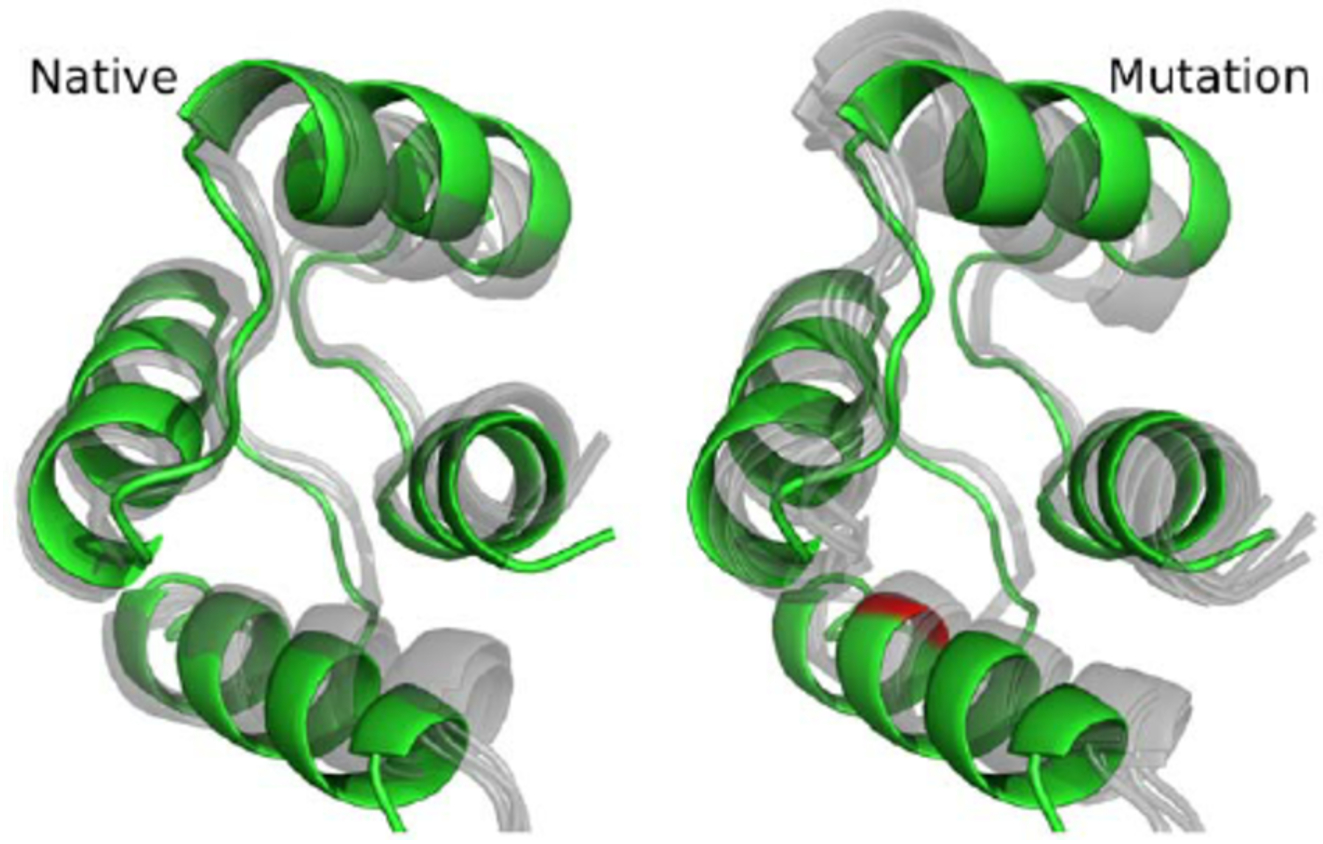
Stratergy 2: Enhancing Protein Resistance to Enzymatic Degradation
Protein degradation is a crucial process in maintaining protein homeostasis within cells, primarily mediated by the ubiquitin-proteasome system (UPS) and the lysosomal pathway. However, excessive protein degradation can lead to cellular dysfunction, as many proteins require stability for a certain period of time after performing their biological functions.
The main factors that influence protein resistance to enzymatic degradation include:
- 1. Surface Charge
- 2. Hydrophobic Surface
- 3. Structural Stability